- Forward osmosis is not dead – interview with leading water consultant Walid Khoury - August 30, 2020
- Listing of major commercial & academic FO players on ForwardOsmosisTech - April 12, 2020
- 0.26MGD FO-SWRO Hybrid for seawater desalination achieves 25% energy reduction compared to MF/SWRO - December 14, 2019
Membrane fouling in reverse osmosis and forward osmosis processes
The difference in membrane fouling between reverse osmosis and forward osmosis processes can be attributed to the underlying driving force for water transport.
In reverse osmosis a hydraulic pressure is applied to overcome the osmotic pressure of the feed solution, thus – in effect – pushing water molecules through the rejection layer of the RO membrane.
In forward osmosis, an osmotic gradient is used to drive water molecule diffusion through the rejection layer of the FO membrane.
In both reverse osmosis and forward osmosis processes, pollutants are retained on the feed side of the rejection layers, causing a build-up of fouling layers over time (see the illustration below). However, due to the lack of hydraulic pressure in the FO process, fouling layers on FO membranes are less compact and therefore easier to remove in subsequent cleaning cycles. This phenomenon has been described in several scientific publications including Mi et. al. 2009 (“Organic fouling of forward osmosis membranes: Fouling reversibility and cleaning without chemical reagents”) and Kim et. al. 2013 (“Combined organic and colloidal fouling in forward osmosis: Fouling reversibility and the role of applied pressure”).
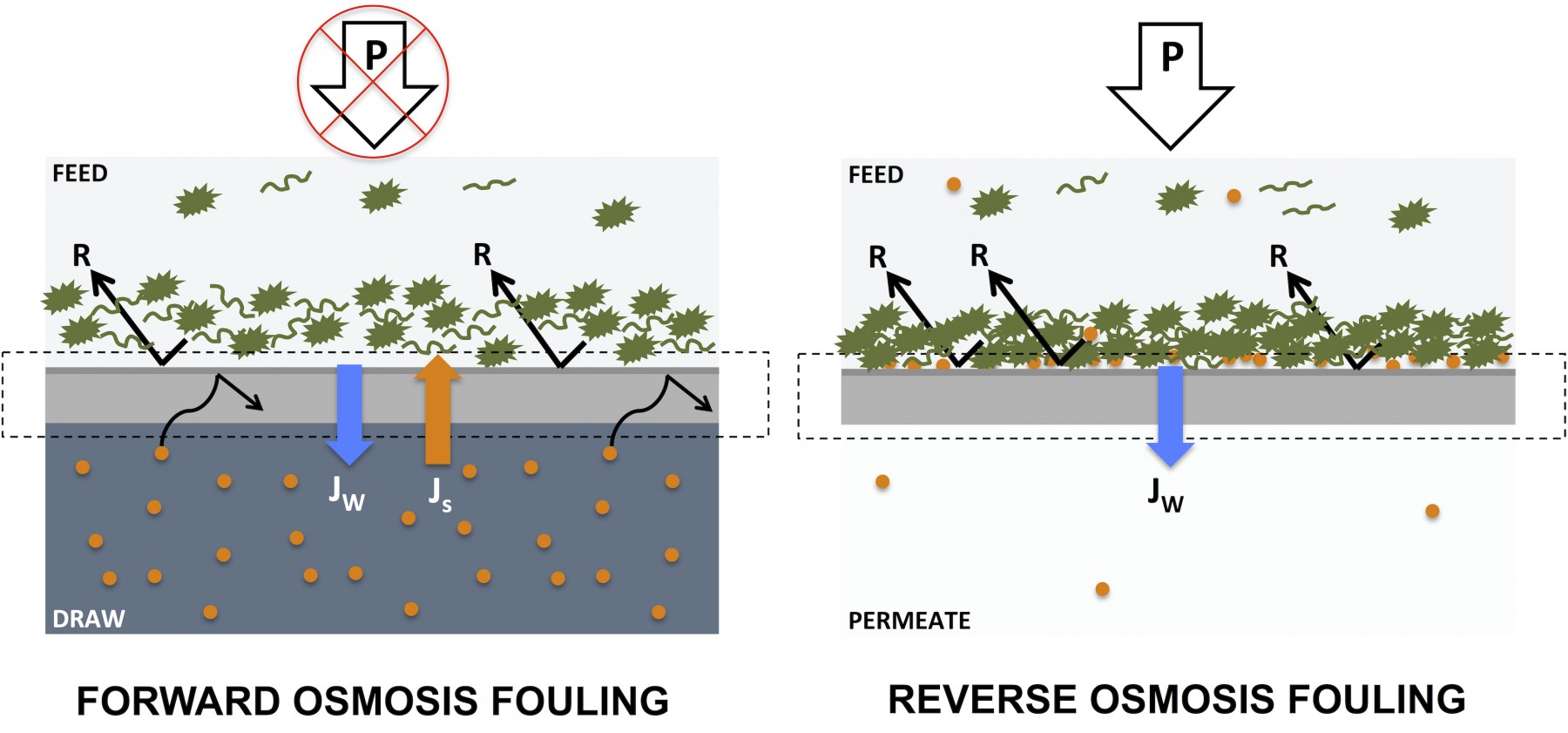
Organic fouling comparison: forward osmosis and reverse osmosis membranes
In their 2009 work, Mi and co-workers used a CTA forward osmosis membrane and feed solutions containing alginate – a model organic foulant – to demonstrate higher cleaning efficiency of the CTA membrane when it was run in forward osmosis mode as compared to reverse osmosis mode. Using a 15 min in situ cleaning process based on running 50mM NaCl through the test chamber at a crossflow velocity of 21 cm/s (i.e. simple physical cleaning based on scouring), CTA membrane performance returned to 99% of baseline performance for forward osmosis mode and only 70% for reverse osmosis mode.
Mi et. al. attributed this reversibility of forward osmosis membrane fouling to the loose structure of the fouling layer and conclude that:
“operating in FO mode may offer an advantage of significantly reducing or even eliminating the need for chemical cleaning (from Mi et. al., 2009)”
Fouling reversibility comparison: forward osmosis and reverse osmosis processes
In related work from 2013, Kim and co-workers investigated the fouling reversibility of combined organic (alginate) and colloidal (silica particles) fouling on a CTA forward osmosis membrane run in forward osmosis and reverse osmosis modes respectively. After fouling runs, membranes were subjected to a 1 h physical cleaning process based on running the original feed and draw solutions from the start of the experiment at an elevated crossflow velocity of 34,44 cm/s (up from 8,61 cm/s during the fouling run). Care was taken to ensure that the water flux across membranes were identical in individual fouling runs when comparing fouling in forward osmosis and reverse osmosis mode respectively.
The researchers found that fouling in forward osmosis mode was fully reversible – with the given physical cleaning process – when either organic or colloidal foulants were used individually. However, for the combination of organic AND colloidal foulants, flux reversibility was reduced to between 80% and 95% depending on the pH of the feed solution. Importantly, when hydrostatic pressure was introduced in reverse osmosis fouling runs, the fouling layers caused more severe flux reduction and COULD NOT be removed with the given physical cleaning process described above.
In line with the findings of Mi et. al., Kim et. al. conclude:
“operation under an osmotic pressure driving force (i.e. FO) results in less fouling and exhibits higher fouling reversibility compared to operation at hydraulic pressure driving force (i.e. RO). The impact of hydraulic pressure is attributed to the formation of more compact and dense fouling later of the deformable organic foulants and organic-colloidal aggregates (from Kim et. al., 2013)